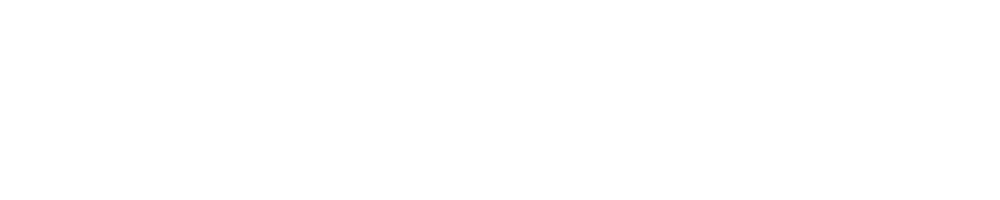
Sonic booms occur when aircraft travel at supersonic speeds and are perhaps the most significant environmental barrier to unrestricted civilian supersonic flight. A NASA research effort focused on understanding and overcoming this barrier, both through the development of approaches to reduce sonic boom noise and to improve understanding of the impact of these reduced noise booms.
Dual room structure equipped with accelerometers and microphones
Existing knowledge of the effect of sonic booms on communities was based primarily on field experiments conducted during the 1960s. It was concluded that high-amplitude sonic booms, such as those from the Concorde, were unacceptable to a large segment of the population, and overland supersonic flights were prohibited.
Recent aeronautic advances have led to technologies that potentially will lower the boom to acceptable levels without serious effects on performance and could lead to a replacement of the current prohibition on civil supersonic overland flights with a noise-based certification criterion. To help inform the regulatory process on the effects of such booms on overflown communities, NASA concentrated its sonic boom research on atmospheric effects, transmission into structures, and human reaction to low-amplitude booms. In order to fully understand human reaction to low-intensity shaped sonic booms, prediction of the transmission of booms into buildings is required, since that is where the majority of people spend most of their time.
This project researched the propagation of impulsive sound such as induced by sonic booms around buildings and thus induced structural loading was investigated both experimentally and numerically. Experiments were conducted on a highly instrumented rectangular building following residential construction practices and exposing it to sonic booms generated by acoustically shaped charges constructed from detonation cord.
The experiments were conducted at Virginia Tech’s Kentland Farm, a facility for agricultural research, which allowed for proper positioning of the test structure and linear charge distributions to replicate sonic boom propagations.
A key objective of this study was to create a realistic sonic boom to excite the structure. The sonic boom was simulated with detonating cord, which is typically used to ignite larger explosives. The replicator consisted of multiple detonating cord strands to create a linear charge distribution. The detonating cord strands were ignited at one end and a boom was then generated in the direction aligned with the detonating cord.
The tested structures were extensively instrumented with microphones and accelerometers to record its vibro-acoustic response. Also, the propagation path between the excitation and the test structure was lined with a series of high-quality microphones provided by NASA. These microphones were used to monitor the pressure wave exciting the structure including propagation effects, such as atmospheric attenuation.
These experiments were then simulated with a three-dimensional numerical model, in the context of geometrical acoustics (GA), by combining an image source method with a method for the diffracted field. This model assumed that the acoustic propagation was linear and that all surfaces were acoustically rigid. The numerical model was then validated against a boundary element (BE) solution as well as the experimental data from the field tests, both showing a good overall agreement.
Ultimately, the validated numerical model was then used by NASA researchers to investigate sound propagation around a cluster of buildings, for a range of elevated source positions simulating sonic booms from aircraft.